Find the volume of the solid of revolution obtained by rotating the region bounded by
about the line
.
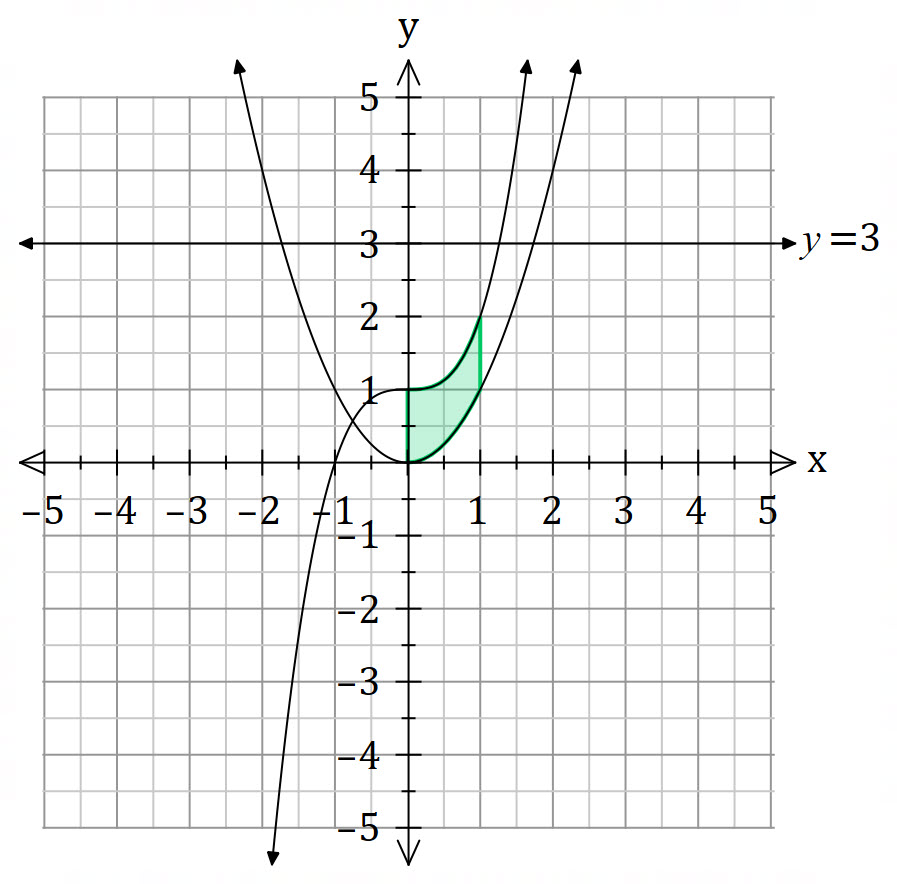

Washer Method
The volume of the solid is the volume of rotated about
subtract the volume of
rotated about
.
is the distance (i.e radius) of the curve and the line.
Shell Method
The shell method is much harder because we need to split the integral into two parts.
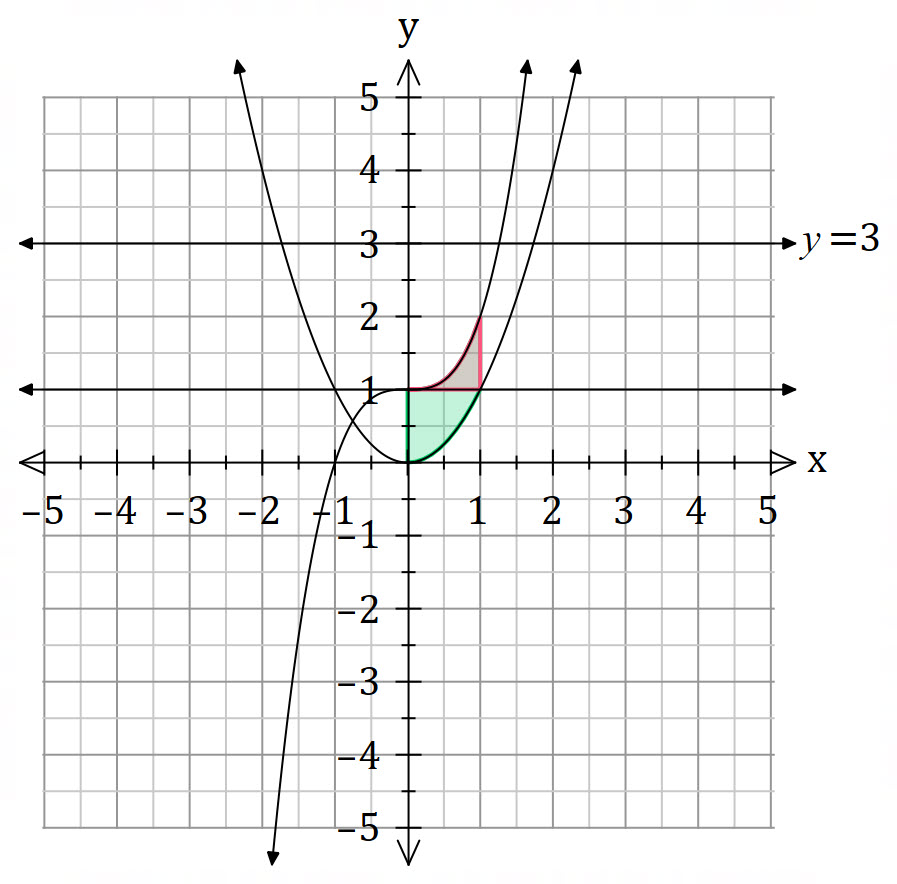

is the distance between each
value and the line of rotation. For example, if we were rotating about the
axis, the distance is
.
is the height of the cylinder between
and
.
is the height of the cylinder between
and
. Refer back to Shell method for more information.
I used a calculator to find this integral
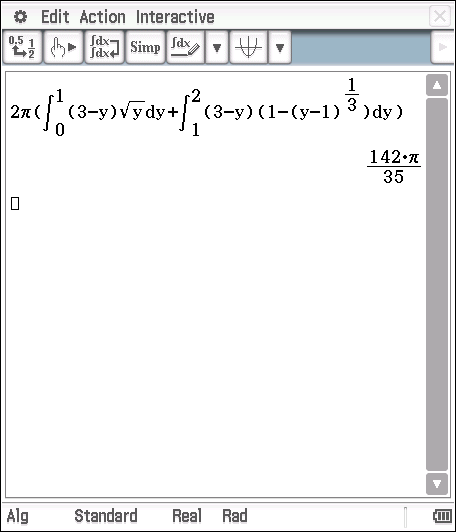